Abstract
Synchrony between basic neuroscience investigations and clinical research has been deficient for quite some time. Translational research includes several dimensions such as laboratory research, clinical demands, government policies, and availability of funds. Through translational research, techniques of neuroanesthesia have become precise and secure over time. Perioperative translational science and contemporary translational research are the two major dimensions of translational research in perioperative neurosciences. The knowledge gap in perioperative neuroscience can be filled with scientific and technological advances with a multidisciplinary approach. In this review, we will discuss various domains of translational research in perioperative neurosciences and have a glance into the translated clinical applications.
Keywords
contemporary translational research, neuroanesthesia, perioperative translational science
Introduction
“Everything that is new has to come out of fundamental research, otherwise it’s not new” Manfred Eigen a Nobel laureate presents his vision, to budding scientific investigators to encourage exploration in fields with scope for investigation. Precisely perioperative neuroscience could be characterized as such a growing field. Although there are evidence-based guidelines for perioperative cardiovascular science, such a potent multidisciplinary framework is lacking for perioperative neuroscience. The knowledge gap in perioperative neuroscience can be filled with scientific and technological advances with a multidisciplinary approach.1 Neuroanesthesiologists are well-positioned to drive relevant clinical and scientific perioperative investigations.
Synchrony between basic neuroscience investigations and clinical research has been deficient for quite some time. As a result, the translation of laboratory outcomes into clinical use is prolonged. Poorly designed clinical trials without a strong basic science background are resource-intense without concrete outcome.2 Translational research includes several dimensions such as laboratory research, clinical demands, government policies, and availability of funds.3 Fruitful translational research demands streamlining of all involved research groups into a translational path. Perioperative translational research in neurosciences is a mechanism to close the gap between the bench and bedside and transfer the molecular basis of neurosurgery and neuroanesthesia into daily medical practice. The two most common types of translational research are—investigator-driven and industry-driven.4 Investigator-driven research is more credible as it does not consider the profit margin of research.5 Investigator-driven research is also a slow process. Whereas industry-driven research is fast through its funding capabilities.6 At the same time, industry-driven research is primarily on topics or products with the potential for profit. In this chapter, we will discuss various domains of translational research in perioperative neurosciences and have a glance into the translated clinical applications. These are summarized in Table 1.7-11
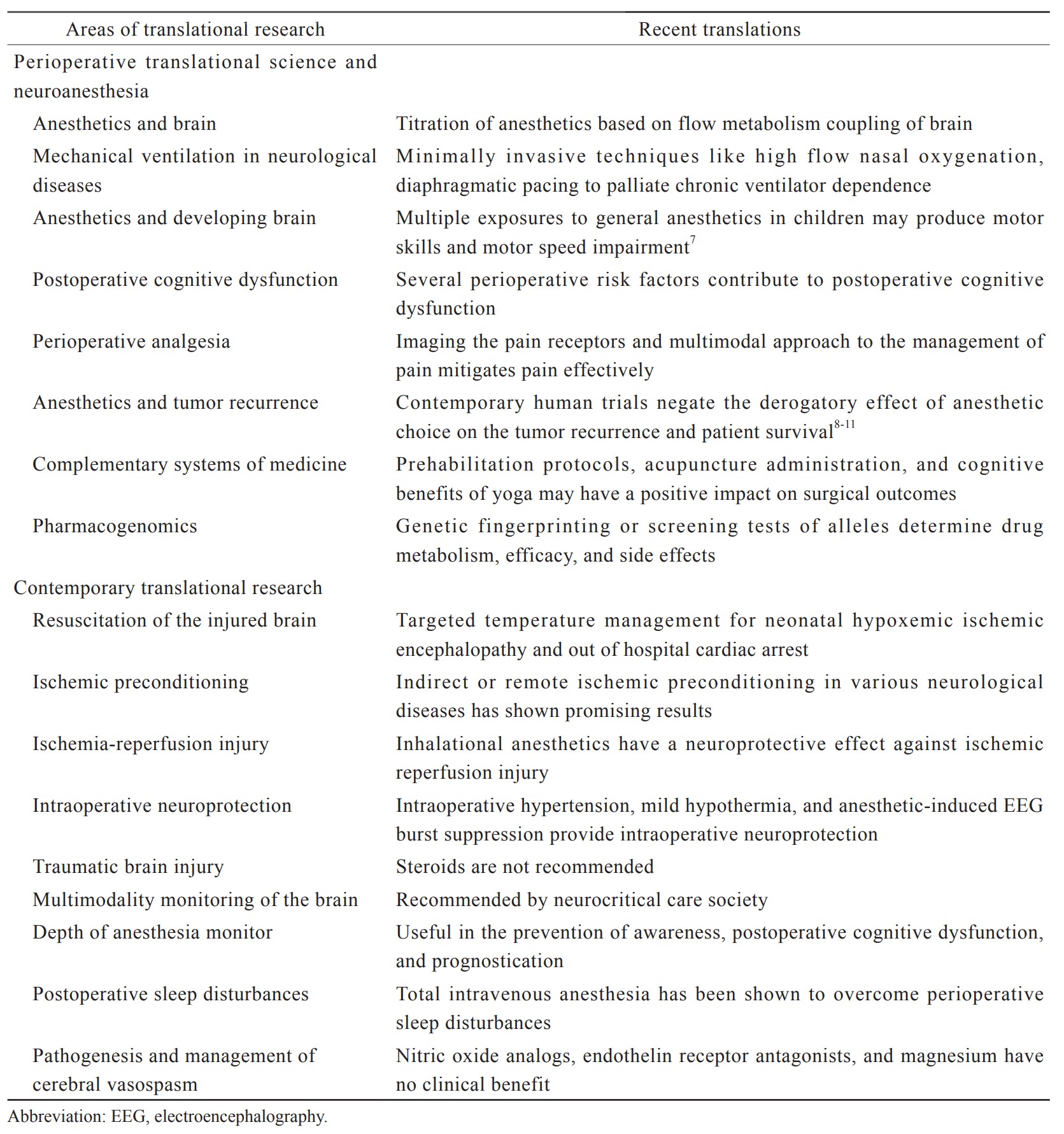
Download full-size image
Perioperative Translational Science and Neuroanesthesia
Research is not new to neuroanesthesia. The concept of neuroanesthesia itself is a result of basic science research that was translated into clinical practice. The invention of neuroanesthesia is a boon to several neurosurgical patients receiving anesthesia and sedation in the operating room or intensive care unit. Through translational research, techniques of neuroanesthesia have become precise and secure over time.12
General Anesthesia and the Brain
Suppression of consciousness by anesthetics has been a mystery for 174 years since the first public demonstration of ether. Anesthetics are known to act on various receptors and channels in the body. They do not simply suspend all brain functions in a widespread manner. Autonomic functions will still be operational when consciousness is lost during general anesthesia.13 Hypothesis of disruption of the frontoparietal network during general anesthesia is increasingly studied.14 When this hypothesis is fully confirmed, this would be an important contribution to translational research. “Communication breakdown theory” between the front and back of the brain, could help us (1) to find out how anesthetic drugs cause unconsciousness and (2) to monitor the effects of anesthetics in the clinical setting.15 Thus, the translational research on brain and anesthetics provides evidence on various pharmacological and physiological aspects of anesthetics like flow-metabolism coupling in the brain, cerebral autoregulation, intracranial pressure, and electrical activity of the brain.16 Such knowledge about anesthetics is essential to plan safe anesthesia for a patient with cerebral pathology.
Precise demonstrations of the exact cerebral pharmacokinetic profile of various anesthetics and adjuvant drugs have enabled the tailoring of clinical practice to suit the needs of patients with a specific diagnosis. A preference for intravenous anesthetics over inhalational anesthetics in a “tight brain scenario” is based on the understanding that flow metabolism coupling is maintained intact in contrast to inhalational anesthetics at a higher minimum alveolar concentration.17 Flow metabolism coupling is the dynamic autoregulation of cerebral blood flow to match the level of cerebral metabolic activity.
The use of succinylcholine and ketamine has long been considered a misfit in neurosurgical patients because of concerns about intracranial hypertension. However, recently published and ongoing human clinical trials demonstrate an acceptable drug profile leaving the primitive insecurities in partial disdain.18,19 Ketamine is now being preferred in various specific arenas namely interference with cerebral infarct expansion in acute ischemic stroke, treatment of drug-resistant depression, adjunct to augment cortical evoked potentials,20 enhancement of electrocorticography recorded seizure activity during epilepsy surgeries, and treatment of neuropathic pain.21,22 This is a classic example of how ongoing and evolving investigations aim to revise previously held opinions to better guide medical practices.
Electroencephalography (EEG)-based demonstration of transition from awake to anesthetized state unique to most anesthetics has helped in the clear delineation of the different progressive stages of hypnosis during general anesthesia.23
Mechanical Ventilation in Neurological Disease
Scientific work done during the polio epidemics on ventilatory support is considered the breakthrough effort in translational research.24 The polio epidemic led to numerous widespread cases of acute respiratory failure, where patients required artificial mechanical support for respiration. This served as a primary driving force for the first invention of mechanical ventilators to assist respiration. Since then, ventilators have evolved from simple negative pressure ventilators to the current era of positive pressure ventilation. Mechanical ventilation of reversible neuromuscular diseases like Guillain-Barre syndrome and myasthenia gravis in the current day neurological practice is credited to this translational research during European polio epidemics.24
Respiratory failure is the most common indication for intensive care unit admission among patients with varied types of neuromuscular diseases. In addition to the traditional practice of administration of mechanical ventilation, newer modes of ventilation with newer non-invasive appliances to support patient’s ventilation must be credited solely to the preclinical studies which have adequately demonstrated their safety and efficacy. Trials continue to be underway to establish their indistinguishable role in the weaning of “the difficult to wean” patients. Clinical practice has seen the use of high flow nasal oxygenation as a minimally invasive and effective adjunct both in the critical care units as well as the operating theatres.25 Diaphragmatic pacing after being deemed successful in animal studies26 has now made its way to palliate “the chronically ventilator-dependent” in clinical practice.27
Anesthetics and the Developing Brain
Development of the nervous system is studied extensively because of concerns about the neurotoxicity of anesthetics to neonatal brains. Evidence to prove the neurotoxic effects of anesthetics is considerably uncertain. Accelerated apoptosis, disrupted synaptogenesis, and cytoarchitecture are the proposed mechanism of neurotoxicity in immature animal brains.28,29 However, implications for the neonatal brain are unknown. Ethical considerations limit the design of such experiments on neonates.
The three hallmark studies in the pediatric cohort evaluating the neurocognitive outcome in pediatric surgical patients are the General Anesthesia or Awake Regional Anesthesia in Infancy
The General Anesthesia compared to Spinal Anesthesia (GAS) Trial,30 Mayo Anesthesia Safety in Kids (MASK) Trial31, and the Pediatric Anesthesia Neurodevelopment Assessment (PANDA) study.32 The GAS trial disproved any significant adverse effect on the intelligence quotient of children at 2 and 5 years after GA for inguinal herniorrhaphy.33 A similar observation was seen in the PANDA study involving healthy children anesthetized before 3 years of age.34 The MASK study could not demonstrate the negative cognitive anesthetic effects on the IQ of children at 3 years post multiple anesthetic exposures. However, a recent analysis of this study by Zaccariello et al.33 found that multiplicity of exposures predisposed patients to greater incidences of motor skill and processing speed impairment.7 The extrapolation of these observations to all patients requires careful reconsideration in the background of different pathologies, duration of anesthesia, and genetic susceptibility.
Postoperative Cognitive Dysfunction
Patients may experience cognitive decline in the postoperative period. As per clinical studies, postoperative cognitive dysfunction (POCD) is defined as a generalized and persistent decline of cognition.35 It does not define which component of cognitive function is impaired. But the preclinical studies focus on definitive neuroinflammation of the hippocampus in the postoperative period.36 Such studies do not explain the generalized postoperative cognitive decline. Thus, for a smooth transition of preclinical research into clinical research of POCD, the effects of surgery on different cognitive functions and brain areas need to be studied.
The role of pre-existing co-morbid illness and high-risk behavior, anesthetic choice, surgical duration, perioperative hemodynamic fluctuations, and perioperative neuro-inflammation have been studied extensively in recent times for the causation of POCD. The correlation of elevated perioperative salivary concentrations of cortisol37 and cerebrospinal fluid monocyte to lymphocyte ratios38 is being tried to better explore options for the prediction of POCD.
Perioperative Analgesia
Interventions to perioperative pain with relevance to current theories of the mechanism of pain are largely unsuccessful.39 Objective pain assessment tools in comatose neurological patients in intensive care units and anesthetized patients are the need of the hour.40 Reliable pain assessment tools need to be developed with appropriate preclinical studies and then translated into clinical practice. Understanding the mechanism of pain in depth may pave the way to diagnosing and treating pain in comatose and anesthetized patients.
Alternative tools for pain assessment like the Nociception Coma Scale41 and the Behavioral Pain Score42 have been developed based on the recent acknowledgment that even a comatose patient can experience pain which is not expressed. Positron emission tomography and functional magnetic resonance imaging of the brain help detect the complex cortical and subcortical network underlying the pain matrix.43 Revisiting and reframing ICU protocols for the periodic assessment and management of pain may contribute to a positive outcome which remains to be elucidated. A multimodal approach to the management of pain continues to be explored in the context of the perioperative phase.44
Anesthetics and Tumor Recurrence
Several studies have shown that anesthetics potentiate the invasion and migration of malignant cells in the lung, breast, and colonic carcinomas.45 Clinical relevance of these findings is not clear at this point of time. However, when the mechanism is understood, such information may be important to choose anesthetics during surgery for malignancy.
Pleiotropism in immunological effects has been demonstrated with different anesthetics.46,47 Amidst studies supporting the association between types of anesthesia and patient survival,48 contemporary human trials negate the derogatory effect of anesthetic choice on the tumor recurrence8,9 and patient survival.10,11
Complementary Systems of Medicine
A variety of alternative systems of medicine have claimed to allay perioperative pain and thus reduce the need for pain medications.49 Such alternative systems, like acupuncture, acupressure, and yoga practices, merit methodical and systematic studies to prove their efficacy.
Alternative forms of therapy through behavioral, lifestyle modifications, and cognitive therapies have been explored for their utility in the perioperative setting. Evaluation of prehabilitation protocols,50 the role of acupuncture administration,51 and application of cognitive benefits of yoga52 may have a positive impact on surgical outcomes. The current status requires well-sampled studies to firmly establish their role in the perioperative setting.
Pharmacogenetics
There is increasing evidence, in recent times, that the anesthetic effects and the susceptibility of an individual are both regulated by genetic polymorphism.53 Genetic make-up determines the extent of anesthetic side effects and adverse drug reactions. Perioperative anesthetic and analgesic management may in the future be guided by the genetic composition of an individual.
The study of pharmacogenetics may eventually lead to genetic “fingerprinting” or screening tests of alleles known to significantly affect drug metabolism, efficacy, and side effects. The effectiveness of neuromuscular blockers such as succinylcholine and mivacurium is strongly associated with genetic factors. Most benzodiazepines are metabolized by hepatic cytochrome P-450 enzymes to more polar metabolites excreted in the bile or urine. Polymorphism exists within cytochrome genes. The diversity of ryanodine receptor (RYR1) polymorphisms associated with malignant hyperthermia triggered by inhalational anesthetics. Genetic variation of the μ-opioid receptor is responsible for variable analgesic response.53
Contemporary Translational Research
The various domains of ongoing translational research are summarized in Figure 1.
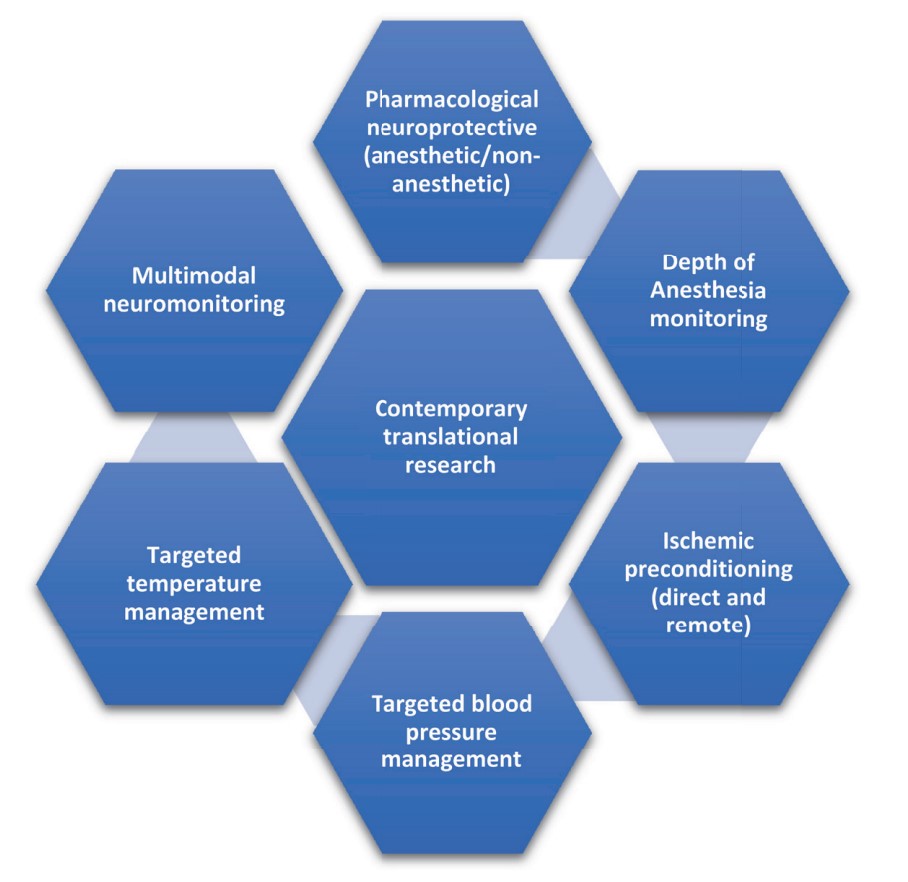
Download full-size image
Resuscitation of the Injured Brain
Cerebral injury or ischemia and cerebral protection are two important dimensions of research in neurosciences. Standard models of injured brain for research have been global and focal ischemia of the brain.54 Pharmacological and non-pharmacological cerebral protection are standard modes of intervention. Pharmacological interventions involve anesthetic drugs and non-pharmacological hypothermia.
Anesthetics: The theory of decrease in cerebral metabolism by anesthetics is the basis for their use in cerebral ischemia.55 A decrease in cerebral metabolic rate of oxygen by 50%–60% at a clinically relevant concentration of anesthetic appeared promising to salvage the ischemic brain. Experimental use of anesthetics for cerebral protection in primate models of global and focal cerebral ischemia supported the theory of pharmacological brain protection. Inspired by these experimental results, large-scale clinical trials were conducted. The use of barbiturates in clinical trials of cardiac arrest, stroke, traumatic brain injury (TBI), and cardiopulmonary bypass has been futile.56 Thus, experimental studies could not be translated to clinical utility. The majority of these studies could not be replicated and had disappointing results. The outcome was an enormous loss of time and resources. Consistency in results of basic sciences research is essential before undertaking large clinical trials.
The use of mild to moderate hypothermia has been also a futile exercise in neurosciences.57 However, profound hypothermia has long established its benefits in complex cardiac surgery. Although experimental research on mild to moderate hypothermia in animals has shown favorable outcomes, their translation into clinical practice for the management of TBI and subarachnoid hemorrhage was not successful. The benefits of hypothermia have been limited presently to post-cardiac status.
Trials of various molecules, namely superoxide dismutase, tirilazad, selfotel, etc, also miserably failed in translation into clinical practice.
Evidence-based support for the practice of Targeted temperature management (TTM) exists as of now for two categories of brain injury: (1) neonatal hypoxic-ischemic encephalopathy; (2) out of hospital cardiac arrest.58
Ischemic Preconditioning
A variety of preclinical data demonstrated the utility of ischemic preconditioning (IPC) in decreasing cerebral ischemia. IPC is effective in both models of focal and global cerebral ischemia. Different IPC stimuli have been used. Some evidence showed that a transient ischemic attack is effective in reducing brain injury during future ischemic strokes.59 However, in contrast to the abundance of preclinical data, few people understood the influence of IPC on cerebral ischemia in humans. Ongoing trials examined the effects of IPC on the brain but no clinical data strongly supported the use of IPC for cerebral protection.
This type of non-pharmacological neuroprotection technique has been demonstrated with clinical utility and effectiveness in varied cerebral pathologies. Direct IPC in carotid artery stenting60 and aneurysmal clipping61 have shown to improve ischemic tolerance to subsequent ischemia-producing events. Preliminary studies of indirect or remote IPC (RIPC) in TBI, cervical spine injury, stroke, neurosurgical patients, and patients with subarachnoid hemorrhage have shown promising results.62,63 Ongoing randomized controlled trials have been proposed to investigate these findings. While we await the results of these studies, the interim results of the ERVAS trial have shown a probable favorable outcome at 6 months post ictus.64 Similar studies are ongoing among the neurosurgical population. The contemporary scenario forces one to view this as an equivocal state in terms of benefit versus futility. Future larger randomized trials can help further clarify these uncertainties.
Ischemia-Reperfusion Injury
A huge number of attempts to translate successful therapeutic strategies for preventing neural ischemia-reperfusion injury (IRI) have failed. Many translational approaches have been unsuccessful because of the use of poor experimental models, clinical testing of inappropriate therapies, and suboptimal clinical trial design. However, due to the intricacy of IRI, only a few pharmacological treatments have been tested in clinical human trials. Caspase inhibitors, P-selectin antagonists, or antioxidant compounds have been tried to limit IRI during organ transplantation, but are mostly unsuccessful to substantiate the hypothesis.
The neuroprotection techniques in stroke target the unintended exacerbation of cellular dysfunction in response to cerebral blood flow restoration to previously ischemic areas.65
Intraoperative Neuroprotection
Pathophysiological mechanisms and therapy of injured brains in the intraoperative period have opened a vast scope of work on intraoperative neuroprotection. The mechanisms presently under research are apoptosis, inflammation, and necrosis. Translational research on intraoperative neuroprotection may help protect the brain from perioperative insults.
Neurosurgical interventions can themselves be a source of focal or global cerebral ischemia. The role of non-pharmacological neuroprotection through the maintenance of systemic and cerebral homeostasis is well known. Recent studies in the anesthetic management of patients with ischemic stroke have re-defined the hemodynamic thresholds to favorable outcomes.67 Intraoperative fall in the mean arterial pressure by more than 40% from baseline was found to be an independent predictor of grave outcomes.
Intraoperative hypertension during the temporary vessel occlusion stage of cerebrovascular surgeries aims at flow-metabolic coupling by transient suppression of cerebral metabolic rate.
A recent meta-analysis including the Intraoperative Hypothermia for Aneurysm Surgery Trial in aneurysmal clipping, craniotomy for TBI, and decompressive craniectomy in malignant ischemic stroke showed a lack of benefit of mild hypothermia over normothermia for neuroprotection.68 However, deep hypothermic circulatory arrest in the anesthetic management of giant cerebral aneurysms may cause amelioration of postoperative neurological deficits.
Intentional suppression of cerebral metabolism by EEG burst suppression using a titrated bolus of anesthetics is an example of pharmacological neuroprotection during cerebrovascular surgeries in vogue in most centers.69
TBI
TBI in humans presents as a gamut of progressive neuropathologies with varying degrees of contusions, hemorrhage, ischemia, brain swelling, and diffuse axonal injury.70 This complex heterogeneity prohibits the development of relevant preclinical TBI models and further translation into clinical practice. Also, primary cerebral injury initiates secondary injury which again depends on the type of primary injury. Thus, the creation of a preclinical TBI model is a complex process. Historically the models have been focal injuries like contusion, laceration, and hemorrhage or diffuse axonal injury. Preclinical animal models are also popular to study and monitor cerebral physiology like cerebral perfusion pressure, cerebral autoregulation, and cerebral blood flow in TBI. However, the translation to clinical practice was limited. Brain-heart cross-talk in TBI has been acknowledged recently.71 Animal models are created to test the hypothesis that therapeutic approaches limit cardiac dysfunction and improve cerebral perfusion and functional outcome. The results are not satisfactory.
The only level 1 recommendation for the management of TBI is the avoidance of steroids in the management. Propofol is recommended for routine increased intracranial pressure (ICP) control; however, barbiturate coma is reserved for super refractory ICP.72
Multimodality Monitoring of the Brain
It is essential to monitor cerebral oxygenation, cerebral blood flow, cerebral electrical and metabolic activity in the operating room and in the intensive care unit. An ideal monitor would be sensitive, specific, accurate, non-invasive, and inexpensive. Currently, available cerebral monitors are labor-intensive, complex, cumbersome, and expensive.
Multimodality monitoring of the brain involves continuous bedside monitoring of cerebral electrical activity, cerebral oxygenation, and cerebral blood flow. Neurological examination, invasive or non-invasive ICP monitoring along with imaging of the brain encompasses comprehensive MMM. Neurocritical care society strongly recommends that the cost/benefit ratio of MMM should be weighed against the overall priorities for delivering basic health care at individual centers.73
Depth of Anesthesia Monitors
The brain is the target organ for anesthetics, but cerebral function monitoring is not popular in anesthesia practice. In recent years, numeric quantification of the depth of anesthesia (DOA) has been devised. But numerous questions were raised about these monitors of DOA. Hence, to acquire objectivity in the prevention of intra-operative awareness needs extensive translational research. Such research is also essential as there is evidence of linking DOA with postoperative poor outcomes.74
Prevention of awareness and consequent psychiatric morbidities is a prime concern in emergency major surgeries performed under general anesthesia. Depth of anesthesia monitoring using raw EEG and its processed variants has an impact on the incidence of POCD.75 Bispectral index (BIS) reduces the anesthetic requirement, recovery time, stay in postanesthesia care unit, and incidence of postoperative nausea and vomiting.76,77 These monitors also help prognostication in post anoxic encephalopathy after cardiac arrest.78 Maintenance of optimal DOA also reduces perioperative morbidity and mortality.79
Postoperative Sleep Disturbances
Rat studies have been conducted to understand sleep mechanisms under general anesthesia, risk factors for postoperative sleep disturbances, harmful effects of postoperative sleep disturbances, and measures to improve or prevent postoperative sleep disturbances.80 Translation to human research is underway but without any concrete and meaningful results. Both pharmacological and non-pharmacological interventions have been experimented upon to prevent postoperative sleep disturbances without much value addition.
General anesthesia alters the sleep architecture in the postoperative period, especially in elderly patients. Sleep disturbances may produce POCD and cardiovascular events. Nonpharmacological treatments with cognitive behavioral therapy and the application of electroacupuncture technology are being investigated.81 Intraoperative use of total intravenous anesthesia has been shown to overcome perioperative sleep disturbances.82
Pathogenesis of Cerebral Vasospasm
Intense preclinical research in the last thirty years has described several molecular mechanisms that possibly play a role in cerebral vasospasm. Both calcium-dependent and calcium-independent mechanisms have been suggested. Translational research in the field of vasospasm is being conducted using a combination of cerebral microdialysis, proteomics, lipidomics, and magnetic resonance spectroscopy. However, clinical translation is unsuccessful at this point of time.
Mechanism of vasospasm as an imbalance in the levels of vasoconstrictor and vasodilatory effects can guide one to explore the spectra of pharmaceuticals that either inhibit the former or enhance the latter. The role of nitric oxide analogs, endothelin receptor antagonists, and magnesium among various other drugs has been studied earlier proving no clinical benefit.83-85
Conclusions
Anesthesiologists are translating themselves as perioperative scientists. The overlap of human physiology and pathology sets an ideal den for translational research in the perioperative environment. Any perioperative morbidity lies within reach of anesthesiology research. Clinical data from operating rooms and critical care units must be hitched with genetic, immunologic, and other preclinical information to transform biomedical science. Since its arrival, anesthesia revolutionized perioperative science with immense potential to translate basic research into clinical practice.
Conflict of Interest
None.
References
1 |
Vlisides PE.
Perioperative neuroscience: a framework for clinical and scientific advancement.
Br J Anaesth. 2019;123(2):107-111.
|
2 |
Rubio DM, Schoenbaum EE, Lee LS, et al.
Defining translational research: implications for training.
Acad Med. 2010;85(3):470-475.
|
3 |
Fleming ES, Perkins J, Easa D, et al.
The role of translational research in addressing health disparities: a conceptual framework.
Ethn Dis. 2008;18(2 Suppl 2):S2-155-160.
|
4 |
Volk HD, Stevens MM, Mooney DJ, Grainger DW, Duda GN.
Key elements for nourishing the translational research environment.
Sci Transl Med. 2015;7(282):282cm2.
|
5 |
Suvarna VR.
Is there a need for investigator-initiated research?
Perspect Clin Res. 2017;8(2):55-57.
|
6 | |
7 |
Sun LS.
Introduction to “Anesthesia and neurodevelopment in children”: a supplement from the Sixth Pediatric Anesthesia Neurodevelopmental Assessment (PANDA) Symposium.
J Neurosurg Anesthesiol. 2019;31(1):101-102.
|
8 |
Ash SA, Buggy DJ.
Does regional anaesthesia and analgesia or opioid analgesia influence recurrence after primary cancer surgery?
Best Pract Res Clin Anaesthesiol. 2013;27(4):441-456.
|
9 |
Heaney A, Buggy DJ.
Can anaesthetic and analgesic techniques affect cancer recurrence or metastasis?
Br J Anaesth. 2012;109(Suppl 1):I17-I28.
|
10 |
Cata JP, Hagan KB, Bhavsar SDO, et al.
The use of isoflurane and desflurane as inhalational agents for glioblastoma surgery.
J Clin Neurosci. 2017;35:82-87.
|
11 |
Dong J, Zeng M, Ji N, et al.
Impact of anesthesia on long-term outcomes in patients with supratentorial high-grade glioma undergoing tumor resection: a retrospective cohort study.
J Neurosurg Anesthesiol. 2020;32(3):227-233.
|
12 |
Khan FA.
Translational research and anesthesia.
J Anaesthesiol Clin Pharmacol. 2014;30(2):151-152.
|
13 |
Hudetz AG.
General anesthesia and human brain connectivity.
Brain Connect. 2012;2(6):291-302.
|
14 |
Lee U, Ku S, Noh G, Baek S, Choi B, Mashour GA.
Disruption of frontal-parietal communication by ketamine, propofol, and sevoflurane.
Anesthesiology. 2013;118(6):1264-1275.
|
15 |
Mashour GA.
General anesthesia and the cortex: communication breakdown?
Anesthesiology. 2019;130(4):526-527.
|
16 |
Slupe AM, Kirsch JR.
Effects of anesthesia on cerebral blood flow, metabolism, and neuroprotection.
J Cereb Blood Flow Metab. 2018;38(12):2192-2208.
|
17 |
Zuleta-Alarcón A, Castellón-Larios K, Niño-de Mejía MC, Bergese SD.
Total intravenous anaesthesia versus inhaled anaesthetics in neurosurgery.
Colomb. J. Anesthesiol. 2015;43(Suppl 1):9-14.
|
18 |
Clancy M, Halford S, Walls R, Murphy M.
In patients with head injuries who undergo rapid sequence intubation using succinylcholine, does pretreatment with a competitive neuromuscular blocking agent improve outcome?
Emerg Med J. 2001;18(5):373-375.
|
19 |
Bowles ED, Gold ME.
Rethinking the paradigm: evaluation of ketamine as a neurosurgical anesthetic.
AANA J. 2012;80(6):445-452.
|
20 |
Sangeetha RP, Bharadwaj S.
KetaDex: a saviour for intraoperative multimodal neurophysiological monitoring in complex neurosurgeries.
Neurol India. 2021;69(1):187-189.
|
21 |
Bar-Joseph G, Guilburd Y, Tamir A, Guilburd JN.
Effectiveness of ketamine in decreasing intracranial pressure in children with intracranial hypertension, J Neurosurg Pediatr.
J Neurosurg Pediatr. 2009;4(1):40-46.
|
22 |
Luthra A, Rath GP.
Ketamine: a neuroanesthesiologist’s friend or foe?
J Neuroanaesth Crit Care. 2018;5(2):77-82.
|
23 |
Purdon PL, Sampson A, Pavone KJ, Brown EN.
Clinical electroencephalography for anesthesiologists: part I: background and basic signatures.
Anesthesiology. 2015;123(4):937-960.
|
24 |
West JB.
The physiological challenges of the 1952 Copenhagen poliomyelitis epidemic and a renaissance in clinical respiratory physiology.
|
25 |
Kim HJ, Asai T.
High-flow nasal oxygenation for anesthetic management.
Korean J Anesthesiol. 2019;72(6):527-547.
|
26 |
Assouad J, Masmoudi H, Gonzalez-Bermejo J, et al.
Diaphragm pacing after bilateral implantation of intradiaphragmatic phrenic stimulation electrodes through a transmediastinal endoscopic minimally invasive approach: pilot animal data.
Eur J Cardiothorac Surg. 2012;42(2):333-339.
|
27 |
Le Pimpec-Barthes F, Legras A, Arame A, et al.
Diaphragm pacing: the state of the art.
J Thorac Dis. 2016;8(Suppl 4):S376-S386.
|
28 |
Lei X, Guo Q, Zhang J.
Mechanistic insights into neurotoxicity induced by anesthetics in the developing brain.
Int J Mol Sci. 2012;13(6):6772-6799.
|
29 |
Bodolea C.
Anaesthesia in early childhood—is the development of the immature brain in danger?
Rom J Anaesth Intensive Care. 2016;23(1):33-40.
|
30 |
Davidson AJ, Disma N, de Graaff JC, et al.
Neurodevelopmental outcome at 2 years of age after general anaesthesia and awake—regional anaesthesia in infancy (GAS): an international multicentre, randomised controlled trial.
Lancet. 2016;387(10015):239-250.
|
31 |
McCann ME, de Graaff JC, Dorris L, et al.
Neurodevelopmental outcome at 5 years of age after general anaesthesia or awake—regional anaesthesia in infancy (GAS): an international, multicentre, randomised, controlled equivalence trial.
Lancet. 2019;393(10172):664-677.
|
32 |
Warner DO, Chelonis JJ, Paule MG, et al.
Performance on the Operant Test Battery in young children exposed to procedures requiring general anaesthesia: the MASK study.
|
33 |
Zaccariello MJ, Frank RD, Lee M, et al.
Patterns of neuropsychological changes after general anaesthesia in young children: secondary analysis of the Mayo Anesthesia Safety in Kids study.
Br J Anaesth. 2019;122(5):671-681.
|
34 |
Lee JJ, Sun LS, Levy RJ.
Report on the Sixth Pediatric Anesthesia Neurodevelopmental Assessment (PANDA) Symposium, “Anesthesia and neurodevelopment in children”.
J Neurosurg Anesthesiol. 2019;31(1):103-107.
|
35 |
Ntalouka MP, Arnaoutoglou E, Tzimas P.
Postoperative cognitive disorders: an update.
Hippokratia. 2018;22(4):147-154.
|
36 |
Cascella M, Bimonte S.
The role of general anesthetics and the mechanisms of hippocampal and extra-hippocampal dysfunctions in the genesis of postoperative cognitive dysfunction.
Neural Regen Res. 2017;12(11):1780-1785.
|
37 |
Han Y, Han L, Dong MM, et al.
Preoperative salivary cortisol AM/PM ratio predicts early postoperative cognitive dysfunction after noncardiac surgery in elderly patients.
Anesth Analg. 2019;128(2):349-357.
|
38 |
Berger M, Murdoch DM, Staats JS, et al.
Flow cytometry characterization of cerebrospinal fluid monocytes in patients with postoperative cognitive dysfunction: a pilot study.
Anesth Analg. 2019;129(5):e150-e154.
|
39 |
Garimella V, Cellini C.
Postoperative pain control.
Clin Colon Rectal Surg. 2013;26(3):191-196.
|
40 |
Pudas-Tähkä SM, Axelin A, Aantaa R, Lund V, Salanterä S.
Pain assessment tools for unconscious or sedated intensive care patients: a systematic review.
J Adv Nurs. 2009;65(5):946-956.
|
41 |
Schnakers C, Chatelle C, Vanhaudenhuyse A, et al.
The Nociception Coma Scale: a new tool to assess nociception in disorders of consciousness.
Pain. 2010;148(2):215-219.
|
42 |
Gomarverdi S, Sedighie L, Seifrabiei MA, Nikooseresht M.
Comparison of two pain scales: behavioral pain scale and critical-care pain observation tool during invasive and noninvasive procedures in intensive care unit-admitted patients.
Iran J Nurs Midwifery Res. 2019;24(2):151-155.
|
43 |
Boly M, Faymonville ME, Schnakers C, et al.
Perception of pain in the minimally conscious state with PET activation: an observational study.
Lancet Neurol. 2008;7(11):1013-1020.
|
44 |
Vadivelu N, Kai AM, Tran D, Kodumudi G, Legler A, Ayrian E.
Options for perioperative pain management in neurosurgery.
J Pain Res. 2016;9:37-47.
|
45 |
Yang W, Cai J, Zabkiewicz C, Zhang H, Ruge F, Jiang WG.
The effects of anesthetics on recurrence and metastasis of cancer, and clinical implications.
World J Oncol. 2017;8(3):63-70.
|
46 |
Huang H, Benzonana LL, Zhao H, et al.
Prostate cancer cell malignancy via modulation of HIF-1α pathway with isoflurane and propofol alone and in combination.
Br J Cancer. 2014;111(7):1338-1349.
|
47 |
Chen J, Ouyang Y, Cao L, et al.
Diazepam inhibits proliferation of human glioblastoma cells through triggering a G0/G1 cell cycle arrest.
J Neurosurg Anesthesiol. 2013;25(3):285-291.
|
48 |
Wigmore TJ, Mohammed K, Jhanji S.
Long-term survival for patients undergoing volatile versus IV anesthesia for cancer surgery: a retrospective analysis.
Anesthesiology. 2016;124(1):69-79.
|
49 | |
50 |
Rivera-Rivera PA, Rios-Lago M, Sanchez-Casarrubios S, et al.
Cortical plasticity catalyzed by prehabilitation enables extensive resection of brain tumors in eloquent areas.
J Neurosurg. 2017;126(4):1323-1333.
|
51 |
Cho SY, Yang SB, Shin HS, et al.
Anti-inflammatory and immune regulatory effects of acupuncture after craniotomy: study protocol for a parallel-group randomized controlled trial [published correction appears in Trials.
Trials. 2017;18(1):481]. Trials. 2017;18(1):10.
|
52 |
Rao RM, Nagendra HR, Raghuram N, et al.
Influence of yoga on postoperative outcomes and wound healing in early operable breast cancer patients undergoing surgery.
Int J Yoga. 2008;1(1):33-41.
|
53 |
Palmer SN, Giesecke NM, Body SC, Shernan SK, Fox AA, Collard CD.
Pharmacogenetics of anesthetic and analgesic agents.
Anesthesiology. 2005;102(3):663-671.
|
54 |
Hossmann KA.
Experimental models for the investigation of brain ischemia.
Cardiovasc Res. 1998;39(1):106-120.
|
55 |
Mrozek S, Vardon F, Geeraerts T.
Brain temperature: physiology and pathophysiology after brain injury.
Anesthesiol Res Pract. 2012;2012:989487.
|
56 |
Safar P, Bleyaert A, Detre K, Moossy J, Reinmuth O.
Cerebral resuscitation with barbiturates.
Anesthesiology. 1986;65(2):234-235.
|
57 |
Ma H, Sinha B, Pandya RS, et al.
Therapeutic hypothermia as a neuroprotective strategy in neonatal hypoxic-ischemic brain injury and traumatic brain injury.
Curr Mol Med. 2012;12(10):1282-1296.
|
58 |
Madden LK, Hill M, May TL, et al.
The implementation of targeted temperature management: an evidence-based guideline from the Neurocritical Care Society.
Neurocrit Care. 2017;27(3):468-487.
|
59 |
Schaller B.
Ischemic preconditioning as induction of ischemic tolerance after transient ischemic attacks in human brain: its clinical relevance.
Neurosci Lett. 2005;377(3):206-211.
|
60 |
Faries PL, DeRubertis B, Trocciola S, Karwowski J, Kent KC, Chaer RA.
Ischemic preconditioning during the use of the PercuSurge occlusion balloon for carotid angioplasty and stenting.
Vascular. 2008;16(1):1-9.
|
61 |
Chan MTV, Boet R, Ng SCP, Poon WS, Gin T.
Effect of ischemic preconditioning on brain tissue gases and pH during temporary cerebral artery occlusion.
Acta Neurochir Suppl. 2005;95:93-96.
|
62 |
Meng R, Asmaro K, Meng L, et al.
Upper limb ischemic preconditioning prevents recurrent stroke in intracranial arterial stenosis.
Neurology. 2012;79(18):1853-1861.
|
63 |
Gonzalez NR, Hamilton R, Bilgin-Freiert A, et al.
Cerebral hemodynamic and metabolic effects of remote ischemic preconditioning in patients with subarachnoid hemorrhage.
Acta Neurochir Suppl. 2013;115:193-198.
|
64 |
Palaniswamy SR, Ramesh VJ, Sriganesh, Christopher R, Bhat D.
A0022 effect of remote ischemic preconditioning on cerebral vasospasm and biomarkers of cerebral ischemia in aneurysmal subarachnoid hemorrhage.
J Neuroanaesth Crit Care. 2019;6(1):S10.
|
65 |
Xiong XY, Liu L, Yang QW.
Refocusing neuroprotection in cerebral reperfusion era: new challenges and strategies.
Front Neurol. 2018;9:249.
|
66 |
Minguet G, Joris J, Lamy M.
Preconditioning and protection against ischaemia-reperfusion in non-cardiac organs: a place for volatile anaesthetics?
Eur J Anaesthesiol. 2007;24(9):733-745.
|
67 |
Simonsen CZ, Yoo AJ, Sørensen LH, et al.
Effect of general anesthesia and conscious sedation during endovascular therapy on infarct growth and clinical outcomes in acute ischemic stroke: a randomized clinical trial.
JAMA Neurol. 2018;75(4):470-477.
|
68 |
Galvin IM, Levy R, Boyd JG, Day AG, Wallace MC.
Cooling for cerebral protection during brain surgery.
Cochrane Database Syst Rev. 2015;1:CD006638.
|
69 |
Beheiry HE.
Protecting the brain during neurosurgical procedures: strategies that can work.
Curr Opin Anaesthesiol. 2012;25(5):548-555 doi:10.
|
70 |
Mckee AC, Daneshvar DH.
The neuropathology of traumatic brain injury.
Handb Clin Neurol. 2015;127:45-66.
|
71 |
Hrishi AP, Lionel KR, Prathapadas U.
Head rules over the heart: cardiac manifestations of cerebral disorders.
Indian J Crit Care Med. 2019;23(7):329-335.
|
72 |
Dash HH, Chavali S.
Management of traumatic brain injury patients.
Korean J Anesthesiol. 2018;71(1):12-21.
|
73 |
Le Roux P, Menon DK, Citerio G, et al.
Consensus summary statement of the International Multidisciplinary Consensus Conference on Multimodality Monitoring in Neurocritical Care: a statement for healthcare professionals from the Neurocritical Care Society and the European Society of Intensive Care Medicine.
Intensive Care Med. 2014;40(9):1189-1209.
|
74 |
Umamaheswara Rao GS.
Translational research in neuroanaesthesia.
J Neuroanaesth Crit Care. 2014;1(1):13-19.
|
75 |
Chan MTV, Cheng BCP, Lee TMC, Gin T, CODA Trial Group.
BIS-guided anesthesia decreases postoperative delirium and cognitive decline.
J Neurosurg Anesthesiol. 2013;25(1):33-42.
|
76 |
Punjasawadwong Y, Phongchiewboon A, Bunchungmongkol N.
Bispectral index for improving anaesthetic delivery and postoperative recovery.
Cochrane Database Syst Rev. 2014;2014(6):CD003843.
|
77 |
Wildes TS, Mickle AM, Ben Abdallah A, et al.
Effect of electroencephalography-guided anesthetic administration on postoperative delirium among older adults undergoing major surgery: the ENGAGES randomized clinical trial.
JAMA. 2019;321(5):473-483.
|
78 |
Hrishi AP, Prathapadas U, Lionel KR, Puthanveedu DK, Sethuraman M.
Anoxic brain injury: the abominable malady.
|
79 |
Petsiti A, Tassoudis V, Vretzakis G, et al.
Depth of anesthesia as a risk factor for perioperative morbidity.
Anesthesiol Res Pract. 2015;2015:829151.
|
80 | |
81 |
Luo M, Song B, Zhu J.
Sleep disturbances after general anesthesia: current perspectives.
Front Neurol. 2020;11:629.
|
82 |
Murphy M, Bruno MA, Riedner BA, et al.
Propofol anesthesia and sleep: a high-density EEG study.
Sleep. 2011;34(3):283-291.
|
83 |
Siuta M, Zuckerman SL, Mocco J.
Nitric oxide in cerebral vasospasm: theories, measurement, and treatment.
Neurol Res Int. 2013;2013:972417.
|
84 |
Macdonald RL, Higashida RT, Keller E, et al.
Randomized trial of clazosentan in patients with aneurysmal subarachnoid hemorrhage undergoing endovascular coiling.
Stroke. 2012;43(6):1463-1469.
|
85 |
Wong GKC, Poon WS, Chan MTV, et al.
Intravenous magnesium sulphate for aneurysmal subarachnoid hemorrhage (IMASH): a randomized, double-blinded, placebo-controlled, multicenter phase III trial.
Stroke. 2010;41(5):921-926.
|