Abstract
Background
Liver surgery is a major abdominal operation associated with a dramatic change in intraoperative hemodynamics; thus, the infusion strategy is challenging for anesthesiologists. Studies have demonstrated that stroke volume variation (SVV) can be used to predict fluid responsiveness during major abdominal surgery. SVV can be used as a guide for the administration of intraoperative fluids to improve postoperative prognosis. In the present study, we planned to investigate whether high- or low-SVV in liver surgery is associated with fewer postoperative complications.
Methods
This study was a prospective randomized trial of 74 patients who underwent hepatectomy. The patients were divided into two groups for SVV-guided infusion during tumor resection surgery using a low-SVV (≤ 10%, n = 37) or high-SVV (> 10%, n = 37) strategy. The primary outcome was postoperative complications, namely infection, pleural effusion, and atelectasis. The secondary outcomes were differences in perioperative physiological variables and postoperative pain.
Results
No differences in postoperative complications within 30 days of surgery were observed between the low-SVV and high-SVV groups. However, we observed lower estimated glomerular filtration rates (eGFRs) and higher alanine transaminase (ALT) levels in the high-SVV group after surgery.
Conclusion
Patients who underwent major liver tumor resection with the low-SVV or high-SVV strategy exhibited no
differences in postoperative complications (48.6% vs. 45.9%;
Keywords
alanine transaminase (ALT), estimated glomerular filtration rate (eGFR), fluid therapy, hepatectomy, stroke volume variation (SVV)
Introduction
Perioperative fluid management considerably affects the prognosis and physiological recovery of patients. Excessive body fluids can impair heart and lung function, prolong the recovery of bowel function, and induce tissue oxidation, which can ultimately prevent wound healing.1 Conversely, volume depletion can lead to microcirculation disorders, which can lead to tissue damage and organ dysfunction.2,3 In clinical settings, major surgery is associated with risks of hypovolemia, blood loss, and massive fluid transfer, which can lead to organ dysfunction, postoperative morbidity, and increased mortality. Central venous pressure (CVP) lacks sensitivity and specificity as a marker.4 Stroke volume variation (SVV) has been demonstrated to be superior to both CVP and cardiac filling pressure as a marker and is associated with improved prognoses and shortened postoperative hospital stays for patients undergoing major surgery.5 Gan et al.6 observed that optimization of volume during surgery through esophageal Doppler monitoring results in earlier recovery of bowel function, a reduced incidence of postoperative nausea and vomiting, and shortened postoperative hospital stays. Su et al.7 and Wakeling et al.8 have suggested that fluid titration with esophageal Doppler monitoring during bowel surgery improves hemodynamic parameters (e.g., cardiac output) and may prevent postoperative complications and reduce length of hospital stay. However, esophageal Doppler monitoring requires training to be effectively utilized.9
Lopes et al.10 and McKendry et al.11 have demonstrated that pulse pressure variation (PPV) during high-risk surgery and cardiac surgery can improve postoperative outcomes and reduce the number of intensive care unit (ICU) admissions and the length of hospital stays. In general, both SVV and PPV are more specific and sensitive for predicting a positive response (increased cardiac output due to fluid stimulation) than cardiac filling pressure or CVP. This may be due to the use of goal-directed fluid management to optimize tissue perfusion, thereby enhancing microcirculation, oxygen perfusion, and postoperative recovery processes.12
Anesthesiologists have debated whether to use lenient or restrictive infusion regimens. Some meta-analyses have suggested the use of restrictive infusion strategies and goal-directed fluid therapy instead of traditional numerically guided infusion using reference vital signs to reduce the incidence of morbidity following colorectal resection.13 Moreover, the SVV-guided infusion strategy with cut-off point of 10 in liver resection surgery is pointed out in a previous study.14 Therefore, we compared infusion management involving low- (≤ 10%) and high-SVV (> 10%) and investigated whether postoperative parameters, including the incidence of complications and amount of blood loss, change depending on SVV-guided strategies in patients undergoing liver tumor resection.
Methods
Study Design
This prospective study was conducted at Kaohsiung Veterans General Hospital in Kaohsiung, Taiwan, from January 1, 2017 to December 31, 2018. The study was approved by the Kaohsiung Veterans General Hospital Institutional Review Board (IRB no. VGHKS16-CT8-25) and was performed in accordance with the tenets of the Declaration of Helsinki (1975) and its later amendments (2013). This trial was also registered on ClinicalTrials.gov (NCT05361252; registration date: April 5, 2022). We initially selected 118 patients requiring hepatectomy. The physiological status of the patients was assessed in terms of American Society of Anesthesiologists scores I–III. The patient exclusion criteria were an extreme body mass index (BMI); age under 20 or over 75 years; emergency surgery; a preexisting cardiac, hepatic, renal, or coagulation disorder; hyperthyroidism; and cardiac arrhythmia. The participants were randomly allocated into two groups (Figure 1) for operation with the low-SVV (≤ 10%, n = 37) or high-SVV (> 10%, n = 37) strategy.
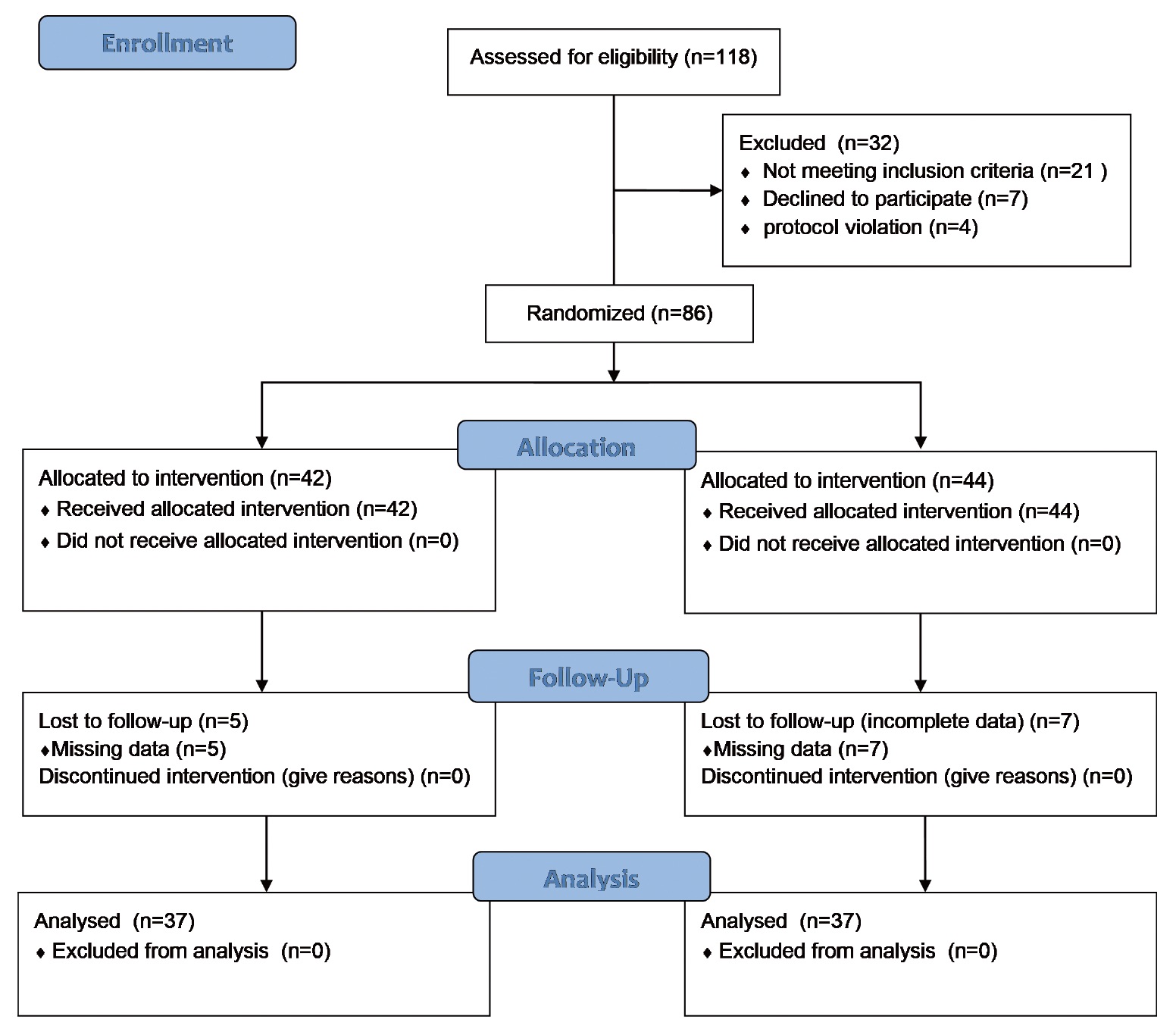
Download full-size image
The primary outcome was the postoperative complication of infection, pleural effusion, atelectasis, bile leakage, pneumonia, massive ascites, and bowel obstruction.
The secondary outcomes were the differences in blood loss, length of hospital stay, and ICU admission. And, it also contained the perioperative physiological variables such as arterial lactate, estimated glomerular filtration rate (eGFR), alanine transaminase (ALT), and hemoglobin. The postoperative pain was assessed with visual analogue scale for three consecutive days.
Sample Size Calculation
The sample size was calculated on the basis of those in other studies,10,15
although most had fewer patients who received an SVV-guided strategy; 18 patients who received an SVV of
less than 10% developed postoperative complications (30.0%), and 35 patients (58.3%;
Randomization and Allocation Concealment
This trial had a randomized study design with simple randomization and a fixed allocation ratio (1:1 to the low-SVV group or high-SVV group). The patients were blinded to the randomization and allocation.
Anesthetic Technique
Standard monitoring techniques, including electrocardiography, pulse oximetry, and end-tidal carbon dioxide (EtCO2), noninvasive blood pressure, and direct arterial blood pressure measurement, were used. Each patient’s EtCO2 level was maintained at 35–45 mmHg, and tidal volume was maintained at 6–8 mL/kg.
Both the low-SVV group and the high-SVV group received general anesthesia (fentanyl, lidocaine, propofol, and atracurium) during the induction period. Intraoperative isoflurane and fentanyl were used during the maintenance phase to ensure the depth of anesthesia and pain relief. When mean arterial pressure (MAP) decreased to below 20% of its initial value, the anesthesiologist would administer an inotrope, such as ephedrine, or a vasopressor, such as norepinephrine, depending on the situation. The restricted infusion period, during which SVV was used as a guide, began at the time of induction and ended with the completion of tumor resection. The infusion period began with the completion of liver tumor resection and ended with the end of anesthesia. During the restricted infusion period, the SVV of the patients in the high-SVV group was maintained over 10%, whereas the SVV of those in the low-SVV group was maintained at or below 10%. When the average arterial pressure decreased to below 20% in either patient group, the anesthesiologist would administer a vasopressor or supplemental fluid depending on the situation. MAP was maintained above 65 mmHg. If the intraoperative hemoglobin level was less than 8 g/dL or if the hematocrit level was less than 30%, a blood transfusion was considered. If the urine output was less than 2 mL/kg/h, the anesthesiologist administered an appropriate infusion or diuretic (Figure 2). Body temperature was maintained at approximately 36°C throughout the operation. Depending on the patient’s intraoperative condition, arterial blood was drawn for blood gas analysis. We divided the entire process into four periods: T1, from the end of induction to the start of operation; T2, from the start of the operation to the start of tumor resection; T3, the tumor resection period; and T4, from the end of tumor resection to the end of anesthesia.
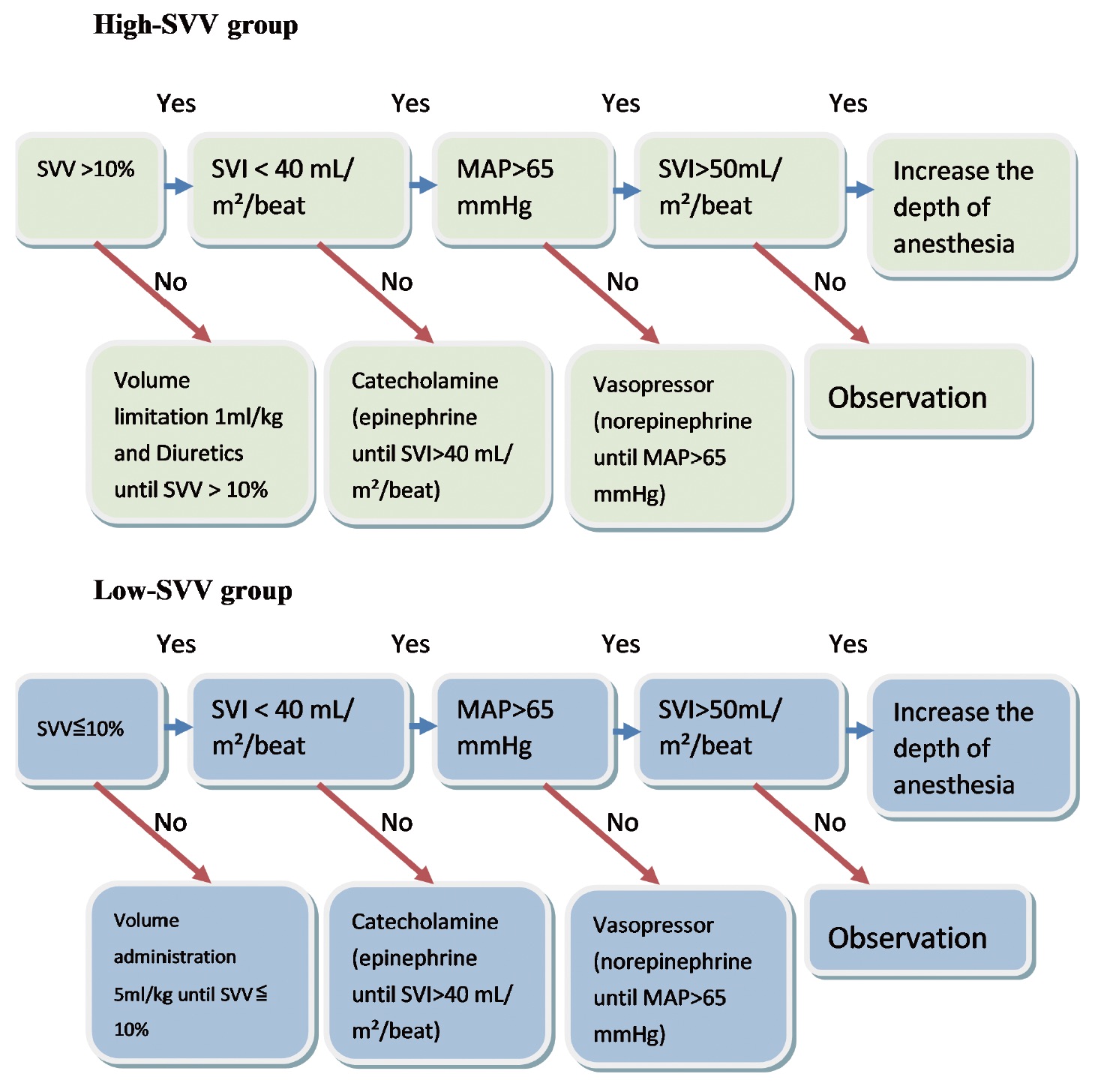
Download full-size image
Abbreviations: MAP, mean arterial pressure; SVI, stroke volume index.
Statistical Analyses
All values are reported as means and standard deviations. Continuous variables were analyzed using two
independent-samples
Results
Patient Characteristics
Table 1 presents the characteristics of the patients in each group. The mean age, BMI, prevalence of hypertension, kidney disease, diabetes, and the history of laparotomy did not differ significantly between the groups. However, the mean number of tumors was significantly greater in the high-SVV group than in the low-SVV group.
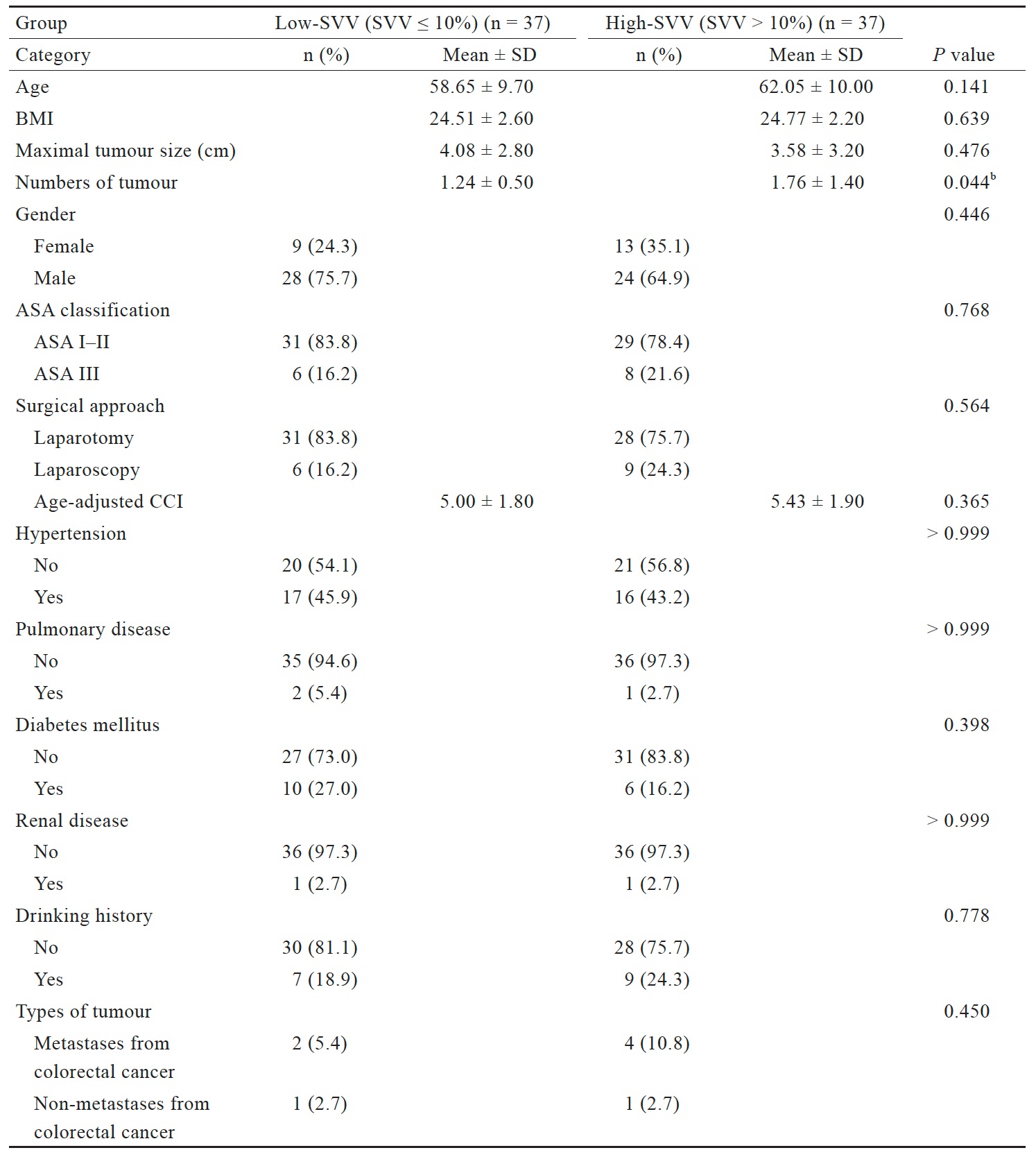
Download full-size image
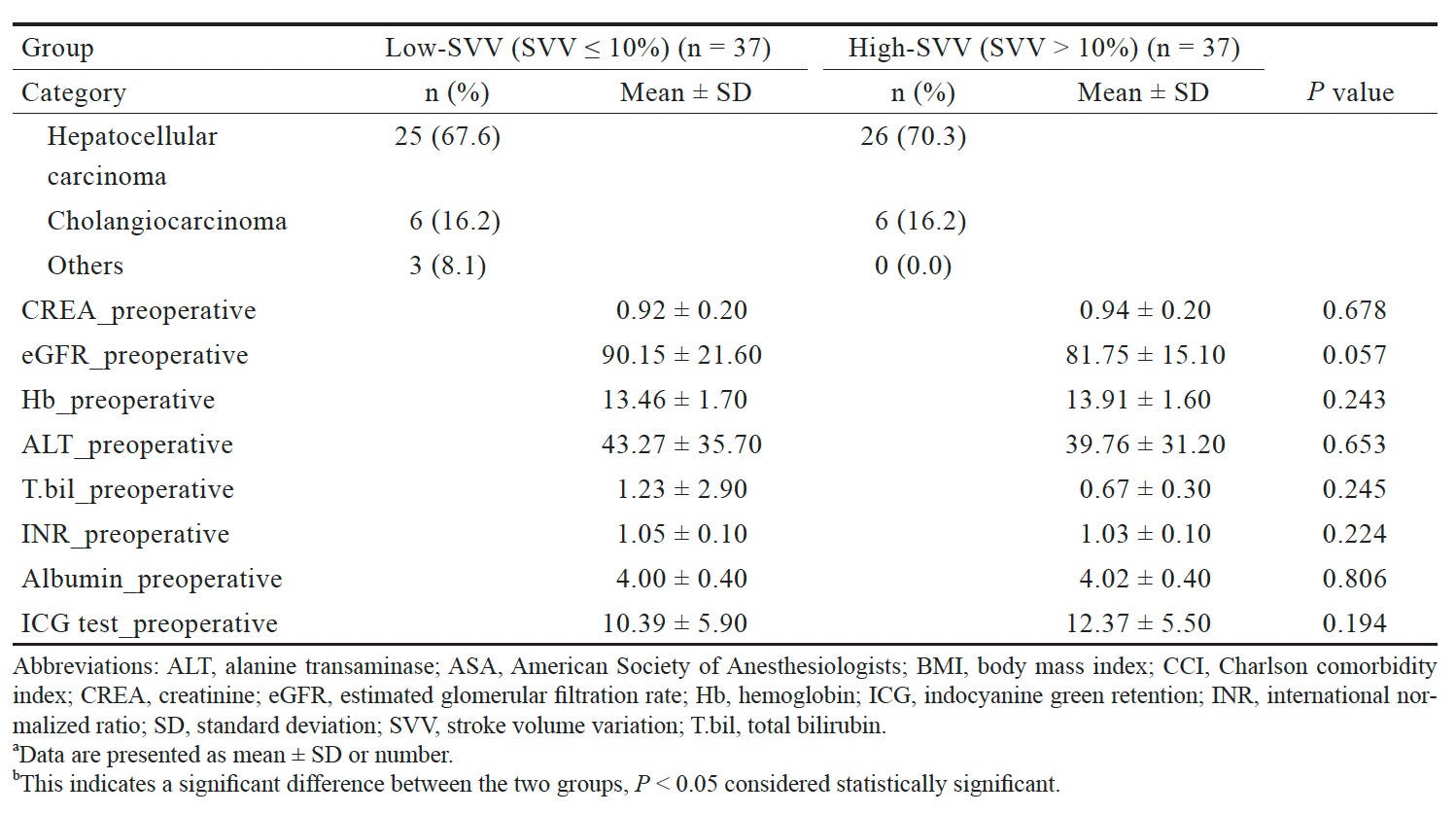
Download full-size image
Perioperative Variables
Table 2 presents the groups’ intraoperative statistics. The surgical times for the low-SVV and high-SVV groups were 252.65 ± 163.58 and 275.46 ± 173.76 min, respectively. Blood loss was greater in the high-SVV group (735.14 ± 639.87 mL) than in the low-SVV group (484.86 ± 438.97 mL), but this difference was not significant. The total intraoperative infusion volume was greater in the high-SVV group (2,436.49 ± 1,134.70 mL) than in the low-SVV group (2,190.5 ± 1,117.1 mL), but this difference was also nonsignificant.
Table 2 also presents the perioperative physiological data of the two groups. The eGFR in the high-SVV group
(79.94 ± 23.37) was significantly lower (
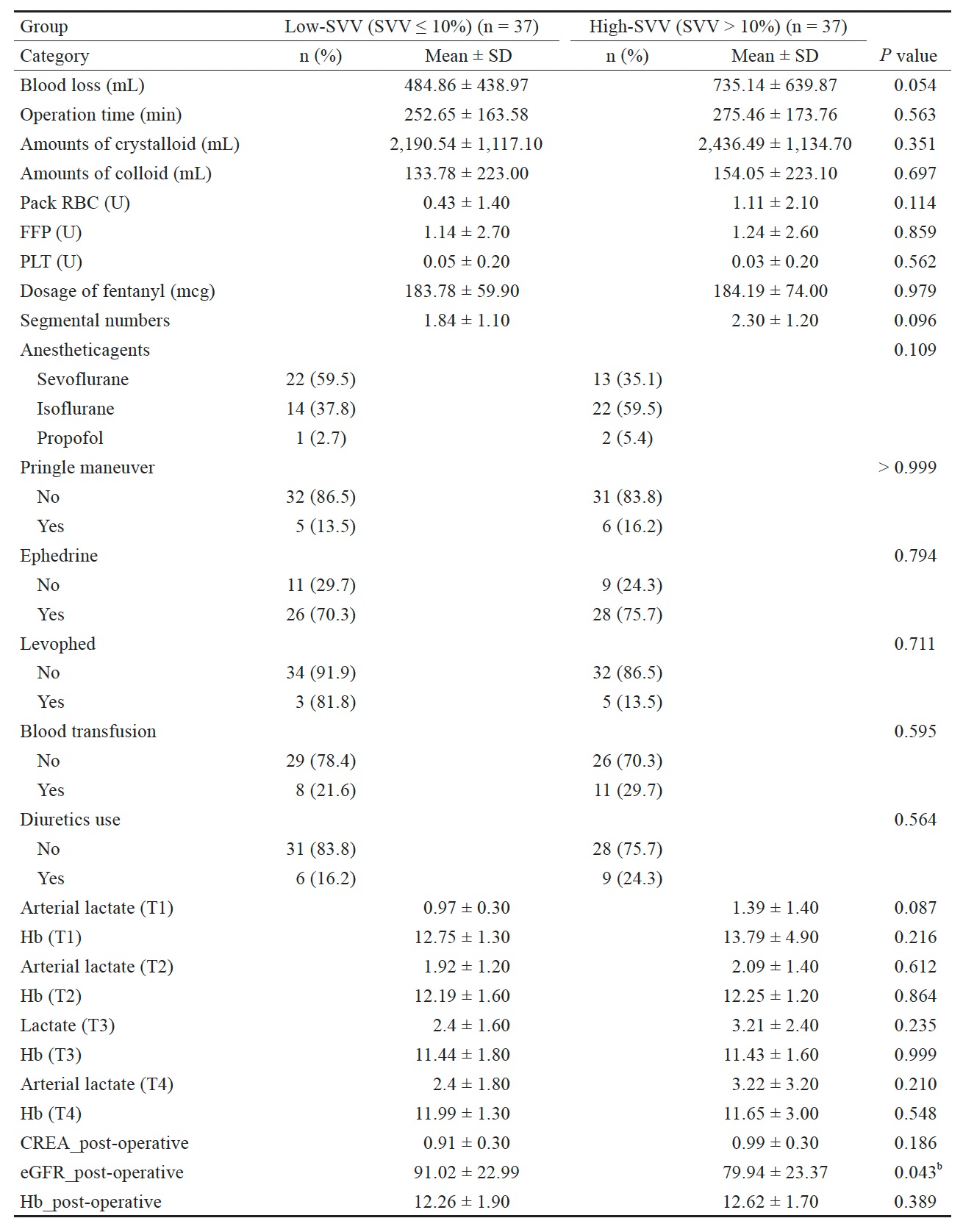
Download full-size image
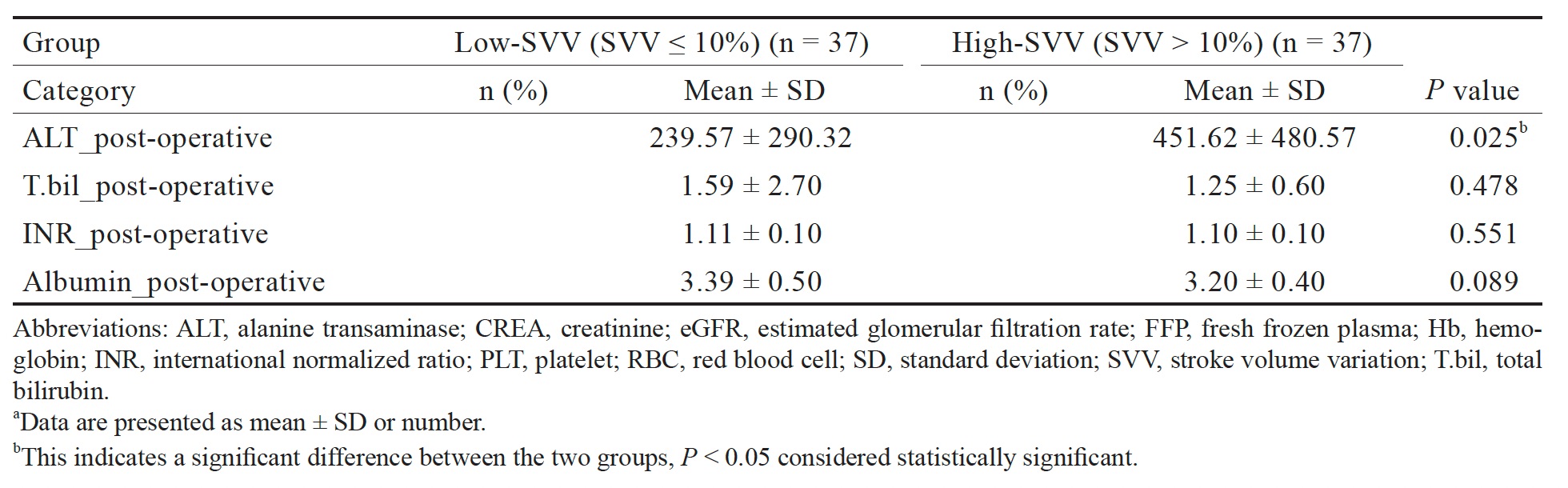
Download full-size image
Postoperative Management and Postoperative Pain
Table 3 presents the postoperative management of the patients. The infusion volume in the postanesthesia care unit for the high-SVV group (451.43 ± 211.95 mL) was not significantly greater than that for the low-SVV group (354.32 ± 208.08 mL). The length of hospital stay in the high-SVV group (13.16 ± 18.97 days) was not significantly different from that in the low-SVV group (11.80 ± 8.23 days). We did not observe significant differences in the duration of catheter usage or the timings of the recovery of normal bowel movements or the resumption of drinking or of eating solid foods. More patients in the high-SVV than in the low-SVV group were transferred to the ICU after surgery, used patient-controlled epidural analgesia (PCEA), and required postoperative blood transfusions or diuretics, but these differences were not statistically significant.
Table 3 also presents the results regarding postoperative pain control. We observed no significant differences between the two groups in the pain scores at rest or during cough or in the PCEA doses from postoperative days 1 through 3. The patient-controlled intravenous analgesia dose on day 1 (102.93 ± 48.50) in the high-SVV group was significantly greater (P = 0.042) than that in the low-SVV group (62.22 ± 27.80). The doses given on days 2 and 3 were not significantly different.
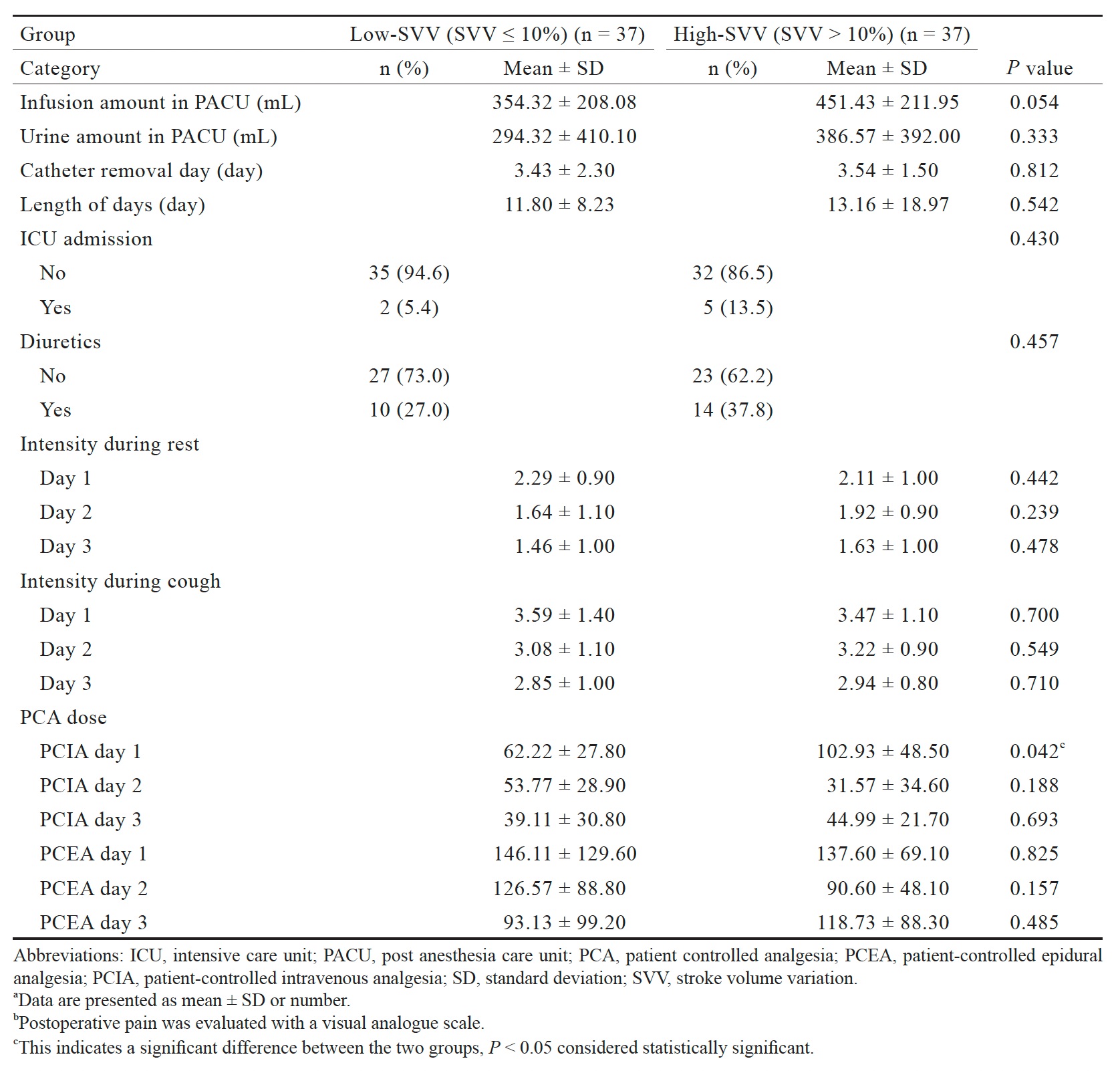
Download full-size image
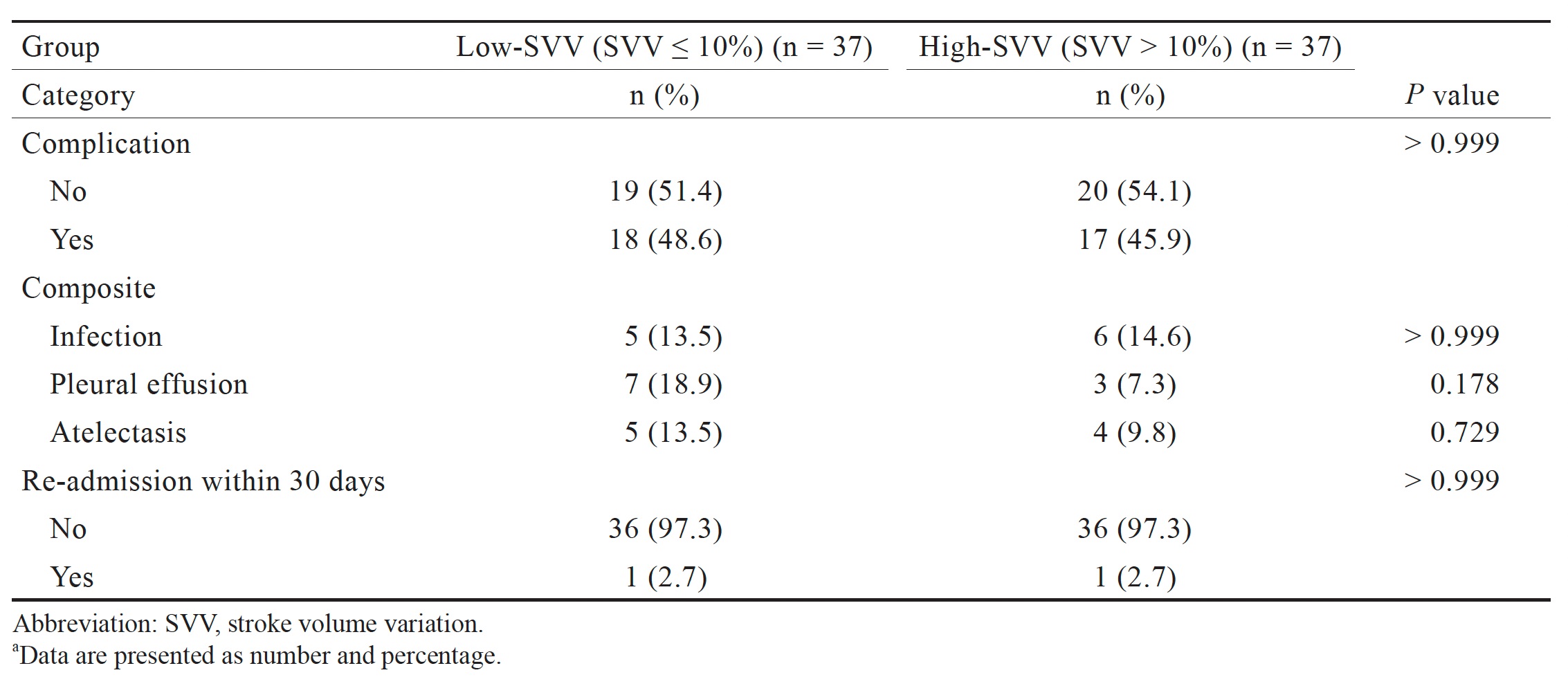
Download full-size image
Postoperative Complications
We observed no significant differences between the two groups in complications or in the number of multiple concurrent diagnoses or readmissions within 30 days after the operation (Table 4).
Discussion
Clinical studies have indicated that an SVV threshold of 10% can be used for determining the appropriate infusion volume for liver transplantation and that a high-SVV strategy can reduce the amount of intraoperative bleeding without increasing the incidence of postoperative complications.14 In addition, compared with using CVP to guide intraoperative infusion for laparoscopic hepatectomy, employing SVV as a guide can reduce the chances of laparoscopic surgery requiring conversion to open surgery, without increasing the risk of complications.8 One study utilized SVV-based infusion guidelines for metastatic liver tumor surgery, and the researchers observed no increase in postoperative complications but a reduction in total intraoperative infusion volume.16 Therefore, we assumed the use of SVV-guided infusion management during hepatectomy to be safe and feasible.
In our study, the differences in postoperative complications between the two groups did not reach statistical significance. This may be the result of maintaining MAP above 65 mmHg during the operations for both groups, which provided adequate organ perfusion for all the patients.
We observed that the total infusion and bleeding volumes were both higher in the high-SVV group, but these results were not statistically significant. We attributed this result to the following factors. First, the number of liver tumors was greater in the high-SVV group than in the low-SVV group, representing a greater challenge for surgeons during tumor resection and thus the likelihood of more bleeding. Second, we included numerous patients with primary liver tumors, whereas other similar studies have included more patients with metastatic tumors; this may explain why we observed no significant reduction in blood loss in the high-SVV group despite other studies reporting significant differences.17
Among the potential complications of hepatectomy, acute kidney injury (AKI) can notably increase the postoperative morbidity and mortality rates. Another study indicated that AKI is a common complication of hepatectomy, but that it is not predictive of impaired long-term survival.18 The same authors also discovered that advanced age, high Model for End-Stage Liver Disease score, major hepatectomy, and prolonged operation were independent predictors of AKI.18 Temporary postoperative deterioration of renal function, similar to that experienced by our patients, has been observed in other studies.19-21 Although multiple factors could affect renal function during the perioperative period,21 increased blood loss during hepatic resection has been associated with a greater risk of AKI.22 Typically, intraoperative CVP is reduced to less than 5 mmHg, and fluids are restricted to reduce blood loss. Postoperative renal function may also be impaired by inadequate perfusion due to the hypovolemic state caused by low-CVP anesthesia.19,23 However, we observed no differences in complications between our two groups; this result may indicate that a SVV-guided infusion strategy is beneficial in liver resection.
Although transaminase is highly concentrated in the liver, increased serum ALT levels indicate liver damage.24 Postoperative serum transaminase levels are independent predictors of postoperative morbidity and mortality and typically peaks during the first 24 hours after surgery;25,26 serum transaminase levels are also markers of hepatocellular injury. Postoperative ALT levels have been significantly correlated with perioperative mortality in patients with posthepatectomy liver failure.27 Peak ALT and aspartate transaminase levels above 812 and 828 U/L ST, respectively, have also been identified as independent predictors of postoperative morbidity and mortality.25,26 In our study, the change in mean ALT level after surgery in the high-SVV group was greater than that in the low-SVV group. Although MAP was maintained above 65 mmHg, the restrictive infusion regimen likely influenced the risk of hepatocellular injury. Moreover, we did not observe any postresection liver failure in our study.
Our study has certain limitations. We did not limit our study to the same type of hepatectomy (e.g., right or left lobectomy); such procedures may differ in blood loss volume and operation time. The number of liver tumors differed significantly between the groups. Surgical complexity may increase the difficulty of identifying an appropriate infusion strategy. Finally, the single-center nature of this study and small sample size are major limitations. Nevertheless, we identified differences between the groups in terms of eGFRs and ALT levels in our secondary outcome analysis. Further research with more participants is required to evaluate the outcomes of different infusion strategies.
Conclusion
In this study, the incidence of complications within 30 days postoperatively did not differ between the low-
and high-SVV groups (48.6% vs. 45.9%,
Acknowledgments
The authors thank CCT for the data collection and analysis sincerely.
Conflicts of Interest
The authors declare no conflicts of interest.
References
1 |
Lang K, Boldt J, Suttner S, Haisch G.
Colloids versus crystalloids and tissue oxygen tension in patients undergoing
major abdominal surgery.
Anesth Analg. 2001;93(2):405-409.
|
2 |
Mythen MG, Webb AR.
The role of gut mucosal hypoperfusion in the pathogenesis of post-operative
organ dysfunction.
Intensive Care Med. 1994;20(3):203-209.
|
3 | |
4 |
Osman D, Ridel C, Ray P, et al.
Cardiac filling pressures are not appropriate to predict hemodynamic response
to volume challenge.
Crit Care Med. 2007;35(1):64-68.
|
5 |
Tavernier B, Makhotine O, Lebuffe G, Dupont J, Scherpereel P.
Systolic pressure variation as a guide to fluid therapy in patients with
sepsis-induced hypotension.
|
6 |
Gan TJ, Soppitt A, Maroof M, et al.
Goal-directed intraoperative fluid administration reduces length of hospital
stay after major surgery.
Anesthesiology. 2002;97(4):820-826.
|
7 |
Su NY, Huang CJ, Tsai P, Hsu YW, Hung YC, Cheng CR.
Cardiac output measurement during cardiac surgery: esophageal Doppler versus
pulmonary artery catheter.
Acta Anaesthesiol Sin. 2002;40(3):127-133.
|
8 |
Wakeling HG, McFall MR, Jenkins CS, et al.
Intraoperative oesophageal Doppler guided fluid management shortens
postoperative hospital stay after major bowel surgery.
Br J Anaesth. 2005;95(5):634-642.
|
9 |
Lefrant JY, Bruelle P, Aya AG, et al.
Training is required to improve the reliability of esophageal Doppler to
measure cardiac output in critically ill patients.
Intensive Care Med. 1998;24(4):347-352.
|
10 |
Lopes MR, Oliveira MA, Pereira VOS, Lemos IPB, Auler JOC Jr, Michard F.
Goal-directed fluid management based on pulse pressure variation monitoring
during high-risk surgery: a pilot randomized controlled trial.
Crit Care. 2007;11(5):R100.
|
11 |
McKendry M, McGloin H, Saberi D, Caudwell L, Brady AR, Singer M.
Randomised controlled trial assessing the impact of a nurse delivered, flow
monitored protocol for optimisation of circulatory status after cardiac surgery
[published correction appears in BMJ.
BMJ. 2004;329(7463):438].
BMJ. 2004;329(7460):258.
|
12 |
Boldt J, Ince C.
The impact of fluid therapy on microcirculation and tissue oxygenation in
hypovolemic patients: a review.
Intensive Care Med. 2010;36(8):1299-1308.
|
13 |
Rahbari NN, Zimmermann JB, Schmidt T, Koch M, Weigand MA, Weitz J.
Meta‐analysis of standard, restrictive and supplemental fluid administration in
colorectal surgery.
Br J Surg. 2009;96(4):331-341.
|
14 |
Choi JM, Lee YK, Yoo H, Lee S, Kim HY, Kim YK.
Relationship between stroke volume variation and blood transfusion during liver
transplantation.
Int J Med Sci. 2016;13(3):235-239.
|
15 |
Benes J, Chytra I, Altmann P, et al.
Intraoperative fluid optimization using stroke volume variation in high risk
surgical patients: results of prospective randomized study.
Crit Care. 2010;14(3):R118.
|
16 |
Correa-Gallego C, Tan KS, Arslan-Carlon V, et al.
Goal-directed fluid therapy using stroke volume variation for resuscitation
after low central venous pressure-assisted liver resection: a randomized
clinical trial.
J Am Coll Surg. 2015;221(2):591-601.
|
17 |
Choi SS, Jun IG, Cho SS, Kim SK, Hwang GS, Kim YK.
Effect of stroke volume variation‐directed fluid management on blood loss
during living‐donor right hepatectomy: a randomised controlled study.
Anaesthesia. 2015;70(11):1250-1258.
|
18 |
Lim C, Audureau E, Salloum C, et al.
Acute kidney injury following hepatectomy for hepatocellular carcinoma:
incidence, risk factors and prognostic value.
HPB (Oxford). 2016;18(6):540-548.
|
19 |
Slankamenac K, Breitenstein S, Held U, Beck-Schimmer B, Puhan MA, Clavien PA.
Development and validation of a prediction score for postoperative acute renal
failure following liver resection.
Ann Surg. 2009;250(5):720-728.
|
20 | |
21 |
Peres LAB, Bredt LC, Cipriani RFF.
Acute renal injury after partial hepatectomy.
World J Hepatol. 2016;8(21):891-901.
|
22 |
Jarnagin WR, Gonen M, Fong Y, et al.
Improvement in perioperative outcome after hepatic resection: analysis of 1,803
consecutive cases over the past decade.
Ann Surg. 2002;236(4):397-407.
|
23 |
Moug SJ, Smith D, Wilson IS, Leen E, Horgan PG.
The renal sequelae of a novel triphasic approach to blood loss reduction during
hepatic resection.
Eur J Surg Oncol. 2006;32(4):435-438.
|
24 |
Giannini EG, Testa R, Savarino V.
Liver enzyme alteration: a guide for clinicians.
CMAJ. 2005;172(3):367-379.
|
25 |
Olthof PB, Huiskens J, Schulte NR, et al.
Postoperative peak transaminases correlate with morbidity and mortality after
liver resection.
HPB (Oxford). 2016;18(11):915-921.
|
26 |
Siu J, McCall J, Connor S.
Systematic review of pathophysiological changes following hepatic resection.
HPB (Oxford). 2014;16(5):407-421.
|
27 |
Yu LH, Yu WL, Zhao T, Wu MC, Fu XH, Zhang YJ.
Post-operative delayed elevation of ALT correlates with early death in patients
with HBV-related hepatocellular carcinoma and post-hepatectomy liver failure.
HPB (Oxford). 2018;20(4):321-326.
|